Where Do Spacecraft Live: How to Choose the Right Satellite Orbit?
If you're planning to launch your space startup, selecting the right satellite orbit is a crucial decision. Orbit selection plays a pivotal role in the success of any space venture.
Colony 88. Astronauts 11 420.
We invite you to join the first professional investment community on AngelList focused exclusively on space tech investments: Beyond Earth Technologies
When launching a satellite, selecting the right orbit is crucial for both technical and economic reasons. In this article, we will explore various orbits and their practical applications.
Marat Airapetian, co-founder of Space Ambition, he served as the orbit expert for the launch of Armenia's first domestic satellite, working in the mission control center and overseeing satellite operations. Today, we have invited him to provide an overview of different orbits to help you better navigate them should you decide to launch your own satellite.
In Which Orbits Do Satellites Fly?
Currently, there are over 10,000 functioning satellites in space and more than 300,000 pieces of space debris. These objects all fly in different orbits. Operating a satellite constellation is becoming commonplace for a growing number of companies. Choosing the right orbit is a critical business decision as it can significantly impact costs related to the size of the constellation, payload requirements, launch frequency, satellite lifespan, and more.
Orbits primarily differ in their shape and location in space. In terms of shape, orbits can be circular (though not perfectly), elongated (elliptical in space terms), or unclosed (for interplanetary missions). Depending on their intended use, satellites can be placed in orbits ranging from a few hundred kilometers to hundreds of thousands of kilometers in altitude. The lower limit of a satellite's flight altitude is determined by Earth's atmosphere. The closer a satellite's orbit is to Earth's surface, the more the atmosphere slows it down. In practice, satellites at an altitude of 200 km will fall out of orbit within a few days without constant course corrections.
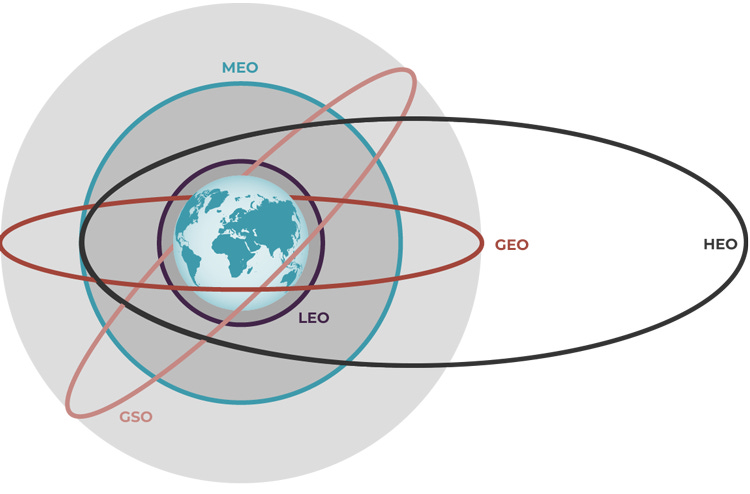
The most widely used orbits are Low Earth Orbits (LEO), located at altitudes up to 2,000 kilometers. Objects in a 400-kilometer orbit, such as the ISS, typically remain in orbit for approximately one year. Despite the common belief that there is no atmosphere in space, tiny atmospheric particles affect flight up to 1,000 km above the surface, which is higher than the orbits of most current satellites. Satellites in orbits above 1,000 km are practically unaffected by Earth's atmosphere and can therefore remain in space for many thousands of years.
Above 2,000 km lies the zone of Medium Earth Orbits (MEO). This region is used by a relatively small number of spacecraft, primarily for research and navigation purposes. For instance, GPS satellites operate at an altitude of 20,350 km with an orbital period of 12 hours. The main challenge in this region is the presence of radiation belts, which contain high-energy charged particles. Radiation in these belts causes satellite electronics to degrade and deteriorate rapidly, making it costly to build satellites that are resistant to such radiation.
Orbits also vary in their inclination relative to the Earth's equator. For example, there are equatorial orbits, where a spacecraft revolves around the Earth in the equatorial plane. There are also polar orbits, where the spacecraft travels perpendicular to the equator along the meridians. Additionally, there are orbits with inclinations between these two extremes.
Geostationary Orbit
The combination of altitude and inclination can produce unique effects. One of the key orbits for communications and broadcasting satellites is the geostationary orbit (GEO). These are equatorial orbits, in the plane of the equator, at an altitude of approximately 36,000 kilometers. At this altitude, the satellite's angular velocity matches the Earth's rotation, making the satellite appear to hover over a single point on the Earth. No other orbit ensures that a satellite remains constantly over the same spot on Earth.
This makes GEO a valuable resource, similar to radio frequencies, and highly convenient for communication and broadcasting satellites. Antennas oriented toward such satellites can maintain stable communication for extended periods, enabling connectivity even in the most remote corners of the globe.
GEO is a unique orbit because it exists only at the equator and at an altitude of 36,000 km. Consequently, the number of satellites that can be placed here is very limited and strictly regulated. Despite not having a large number of satellites, the geostationary orbit is the busiest due to their close proximity. To achieve the geostationary effect, all satellites must be at the same altitude and inclination, distributed along the equator.
Due to this proximity, geostationary orbit is subject to specific regulations. Unlike other orbital regimes, satellite positions in GEO are regulated by the International Telecommunications Union (ITU), a specialized agency of the United Nations. The ITU allocates satellite licenses to member states to prevent harmful interference in the radio-frequency spectrum. A geostationary satellite license from the ITU grants states the right to communicate in specific radio frequency bands from designated positions in the geostationary belt, which they can then allocate to individual operators.
On the downside, placing a satellite in a GEO orbit at 36,000 km altitude requires a significant amount of fuel, making it expensive. Additionally, satellites in such orbits require more powerful transmitters, which further adds to the cost.
Sun-synchronous Orbits
Sun-synchronous orbits (a specific combination of inclination and altitude) are also popular. These orbits are calculated so that a satellite passes over any given point on the Earth's surface at approximately the same local solar time, ensuring consistent lighting conditions. This is particularly advantageous for satellites conducting Earth observation, as the angle of incidence of the sun's rays will be roughly the same on each pass.
Therefore, sun-synchronous orbits are ideal for spacecraft performing tasks such as weather observation, mapping, and Earth surface monitoring. The inclination for sun-synchronous orbits is typically around 97-98 degrees. While a higher inclination is possible, it would require a different orbital altitude and incur additional launch costs.
A satellite's orbit is determined by its velocity and the direction of that velocity. To alter a satellite's altitude, its velocity must be increased or decreased. Conversely, changing a satellite's inclination requires adjusting the direction of its velocity. Notably, altering a satellite's inclination demands significantly more fuel—often dozens of times more—compared to changing its altitude. Therefore, engineers strive to minimize changes in a satellite's inclination throughout its operational lifespan.
Polar Orbits
Polar orbits are also widely utilized—these orbits cause satellites to pass over or very near both poles of the Earth during each revolution. In such orbits, the satellite's orbital plane passes nearly perpendicular to the equator. This configuration enables the satellite to observe the entire surface of the Earth as the planet rotates beneath it. Such orbits also allow to monitor the North and South Pole, melting of glaciers, etc.
Choosing the Right Orbit
Satellites select their orbits based on specific applications. For instance, if designing a satellite for Earth observation, a lower orbit allows the use of a camera with less powerful specifications. Conversely, higher orbits require more powerful cameras. Several factors typically influence orbit selection:
Frequency of coverage over the area of interest (lower orbits result in more visits per day).
Duration of communication windows between the zone and your ground station.
Type of payload (e.g., Earth observation, communication).
For communication and broadcasting satellites, maintaining a stable signal is paramount. Geostationary orbits are ideal because they allow the satellite to remain visible from many points on the Earth's surface without requiring antenna reorientation.
Another approach involves Highly Elliptical Orbits (HEO) with long orbital periods. These orbits are highly elongated, resembling ovals or ellipses, with the most stretched part typically positioned over the Earth's zone of interest. This configuration maximizes the time spent over this zone during each orbit, which can last several hours. As a result, even a few satellites can effectively cover large areas.
An alternative approach to constructing orbits for communication and telecommunication satellites involves low circular orbits. These orbits, at lower altitudes, require less powerful signal amplifiers. However, because each satellite moves rapidly, it covers only a limited area of the Earth's surface. To overcome this limitation, entire networks or "constellations" of many satellites are deployed.
For instance, the Iridium satellite telephone system consists of more than 70 satellites orbiting at approximately 780 km altitude.
Graveyard Orbits
The task of disposing of satellites after their operational life is becoming increasingly urgent due to the proliferation of satellites in space. In 2023, DISH received the first-ever fine from the FCC for failing to de-orbit its EchoStar VII satellite as required by its license terms.
There are two primary methods for satellite disposal: either sending it into the Earth's atmosphere to burn up, or placing it into special disposal orbits. LEO satellites are usually sent into the Earth's atmosphere and GEO satellites are taken to a disposal orbit. It's inefficient for geostationary orbits also sent sattelites to the Earth’s atmosphere due to their distance from Earth.
The designated Graveyard orbit is situated just 200-300 kilometers above geostationary orbit (36,000 km + 200 km). This orbit was chosen to minimize fuel and cost required for satellite disposal. However, this orbit is now populated by hundreds of defunct satellites, potentially posing a future problem. The Kessler syndrome underscores this concern, suggesting that a collision between two objects can trigger a cascade of debris, leading to further collisions.
Perspective Orbits
With the advancement of new technologies, new potential orbits are emerging. Recently, low thrust engines have seen significant development, becoming more affordable and accessible. These engines effectively counteract atmospheric friction, enabling satellites to operate in Very Low Earth orbits (VLEO), such as 250 km. Check out our article about low thrusters here.
Further advancements in engine efficiency have made VLEO at 200 km feasible. As mentioned earlier, at such altitudes, satellites experience significant atmospheric drag. Traditional powerful engines are ineffective in mitigating this effect, but low thrust engines offer a viable solution. VLEO hold promise particularly for Earth observation satellites.
Flying in lower orbits may enable higher resolution images and require less powerful cameras. However, determining efficiency requires research: which is more effective, ultra-low orbits at 200 km with low thrust engines and less powerful cameras, or more conventional orbits at 400 km altitude with standard cameras?
With the advent of Starship, the orbital landscape could shift. The potential drastic reduction in launch costs—potentially lowering the cost per kilogram of payload—could make it easier to deploy satellites into higher orbits. This could lead to a shift in satellite orbital distribution, with companies potentially preferring higher orbits. In theory, this would make it cheaper to launch satellites into higher orbits, and make the satellites themselves more resistant to radiation. But so far, these are just hypotheses. For more insights, you can read our article on Starship's role in the space market.
The study of orbital mechanics is a pivotal aspect of the modern space industry, crucial for determining the success and economic viability of spacecraft. The diverse range of orbits, each with unique features and characteristics, enables satellites to be customized for various missions, including surveillance, communications, scientific research, and navigation. As technologies and mission requirements evolve, orbital mechanics experts continually explore innovative approaches, such as ultra-low orbits, which promise to expand the horizons of future space exploration and utilization.
Forty years ago, calculating a single flight trajectory required dozens of specialists and a week of work. Even today, this topic may seem complex to many. However, with the advancement of technology, anyone with a personal computer can now calculate an orbit. We envision a future where calculating spaceship orbits could become as common as learning mathematics, basic physics, or programming in schools. Perhaps one day, children will study orbital mechanics alongside these subjects. We hope to witness our kids and grandchildren mastering this knowledge as naturally as they do with current school subjects. If you have any ideas to share, please reach out to us at hello@spaceambition.org.