Lunar Resources: Is the Industry Ready for Venture Capital Investment?
The Moon holds Helium-3, gold, and rare earth elements, with 100+ companies racing to take part in the Lunar economy. But is lunar mining truly viable and when is the right time for VCs to bet on it?
Issue 113. Subscribers 39,578.
This post is brought to you by Beyond Earth VC, a US-based early-stage venture firm investing in space tech and deep tech startups.
General Partners Dr. Oleg Demidov and Alex Smolik led the team to spend six months conducting in-depth research on lunar resources to determine whether now is the right time to build a venture-backed business in this field. The award-winning team has a proven track record in this field, having previously won the Mars Colony Prize organized by the Mars Society for their technological model of a sustainable Mars settlement. This model later evolved into the Beyond Earth VC investment thesis, shaping the vision for the future of space ventures.
With so much conflicting information online about lunar resources, we believe this article will provide a fresh, eye-opening perspective. Check out our previous article about investing in Lunar Economy as well - Why We Invested in Lunar Outpost.
This article dives into the previous results, data, achievements, and challenges of lunar resource exploration. We will explore the findings from past missions, discuss the data collected so far—including insights from reports by organizations like the U.S. Geological Survey—and examine the prospects and hurdles of utilizing lunar resources.
By understanding the Moon's resource potential and the progress made thus far, we can better prepare for the next giant leap in space exploration—a leap that promises to transform our relationship with the Moon from that of an observer to a collaborator in the quest for knowledge and sustainable development.
Lunar Resources: What’s Available on the Moon?
Since the 1960s, the Moon has been a focal point of human exploration and scientific inquiry. The Apollo program not only achieved the monumental feat of landing astronauts on the lunar surface but also ignited a global interest in our nearest celestial neighbor. In the ensuing decades, numerous automated missions from countries such as the Soviet Union, Japan, China, India, and collaborations within the European Space Agency have expanded our understanding of the Moon's composition, geology, and potential resources.
These explorations have yielded a wealth of information, revealing that the Moon is more than a desolate landscape of rock and dust. It harbors valuable resources that could be pivotal for future space missions and even have economic implications for Earth. Key among these are:
Water Ice: Confirmation of water ice in permanently shadowed craters at the lunar poles.
Minerals: Mapping of elemental abundances, including iron, titanium, thorium, uranium, and rare earth elements.
Precious Metals: Gold and platinum group metals that can be exported back to Earth to support the lunar economy.
Helium-3: Analysis of regolith samples indicates the presence of helium-3, a potential fuel for future fusion reactors.
Oxygen: Found within lunar minerals like ilmenite, suggesting possibilities for extraction to support life support systems.
As we contemplate a sustained human presence on the Moon and the broader solar system, the utilization of these resources becomes increasingly significant. Building infrastructure on the Moon through In-Situ Resource Utilization (ISRU = process of using local resources) not only reduces the need to transport materials from Earth but also serves as a proving ground for technologies that will be essential for missions to Mars and beyond. Moreover, the concept of an export economy based on lunar resources is gaining traction, presenting new opportunities and challenges.
Past Lunar Missions Highlights
Over the last 50 years there were a number of lunar missions and experiments relevant to resource exploration.
Apollo Program (1969-1972). NASA's Apollo missions were the first and only missions to land humans on the Moon. Astronauts collected 382 kilograms (842 pounds) of lunar rock and soil samples from various locations. These samples provided invaluable information about the Moon's geological composition, revealing the presence of minerals like ilmenite (a source of titanium and oxygen) and offering insights into potential resource extraction methods.
Luna Program (1959-1976). Conducted by the Soviet Union, the Luna Program included the first spacecraft to reach the Moon's surface and return samples to Earth (Luna 16, 20, and 24). These missions analyzed lunar soil, confirming the presence of valuable minerals and contributing to the understanding of the Moon's regolith properties. USSR’s robotic rovers (Lunokhod 1 and 2) have also traversed the lunar surface, analyzing soil mechanics and geology.
Clementine (1994), a joint mission by NASA and the Strategic Defense Initiative Organization, mapped the lunar surface across multiple wavelengths. Data indicated possible water ice in permanently shadowed craters near the lunar poles, sparking interest in lunar water resources for ISRU applications.
Lunar Prospector (1998-1999), NASA spacecraft, orbited the Moon to map its composition and gravitational field, detected hydrogen concentrations at the poles, suggesting the presence of water ice deposits. It provided detailed data on elemental abundances, including potassium, thorium, and uranium.
SMART-1 (2003-2006) was an European Space Agency (ESA) spacecraft that utilized ion propulsion technology to reach the Moon. Performed X-ray and infrared spectrometry to analyze the lunar surface's elemental composition, enhancing knowledge about resources like calcium, aluminum, silicon, magnesium, and iron.
Kaguya (SELENE) (2007-2009) mission was led by Japan Aerospace Exploration Agency (JAXA), and conducted global mapping of the Moon with high-definition cameras and scientific instruments. Provided data on the mineralogy of the lunar surface and mapped gravity anomalies, aiding in the identification of resource-rich areas.
Chandrayaan-1 (2008-2009) by Indian Space Research Organisation (ISRO) was India's first lunar mission. It carried NASA's Moon Mineralogy Mapper (M^3), which detected water molecules and hydroxyl on the lunar surface. Confirmed the widespread presence of water ice in polar regions and mapped various minerals.
Lunar Reconnaissance Orbiter (LRO) (2009-Present) is an operational NASA satellite, equipped with high-resolution cameras and instruments, LRO has been mapping the lunar surface in unprecedented detail. It has identified potential water ice deposits in permanently shadowed regions and provided data crucial for selecting future landing and mining sites.
LCROSS (Lunar Crater Observation and Sensing Satellite) (2009) was NASA’s experimental spacecraft that purposefully crashed into the Cabeus crater near the lunar south pole to analyze the resulting plume. Confirmed the presence of water ice and other volatiles, including hydrogen, ammonia, and methane, highlighting the potential for resource utilization.
Chang'e Program (2007-Present) led by China National Space Administration (CNSA) launched six lunar missions, including Chang’e-6 in 2024, which was humanity's first lunar mission to retrieve samples from the far side of the Moon. Earlier China’s program highlights include:
Chang'e-1 and Chang'e-2: Orbital missions that mapped the Moon's surface and analyzed its composition.
Chang'e-3 (2013): Deployed the Yutu rover, which conducted on-site analyses of lunar soil and rocks, providing data on the Moon's geology and mineral resources.
Chang'e-4 (2019): First mission to soft-land on the Moon's far side, offering unique geological insights and studying mineral composition.
Chang'e-5 (2020): Successfully returned 1.73 kilograms (3.8 pounds) of lunar samples to Earth, the first such retrieval since 1976, allowing detailed laboratory analysis of lunar materials.
Which Resources We Have Found on the Moon and What Are They Good For
Multiple missions described above have gathered huge amounts of data and collectively advanced our understanding of the Moon's resources.
The U.S. Geological Survey's 2022 report on lunar resource exploration provided a detailed overview of current knowledge and future prospects for utilizing the Moon’s resources. The report outlines three key categories of lunar resources: energy, minerals, and water. Solar energy, particularly near the lunar poles, is highlighted as a readily accessible resource due to the high ridges that receive continuous sunlight. Technologies for harnessing solar power are already mature. In contrast, the lunar regolith, which covers the Moon's surface, contains a variety of minerals that can potentially be converted into construction materials and oxygen. However, while technologies to utilize these minerals are in development, they are not yet at a stage where industrial-scale use is feasible.
The USGS has also released a comprehensive digital geologic map of the Moon at a 1:5,000,000 scale, which consolidates previous geologic maps into a unified product. This map includes 43 geologic units categorized into various groups such as crater materials, volcanic formations, and basin structures.
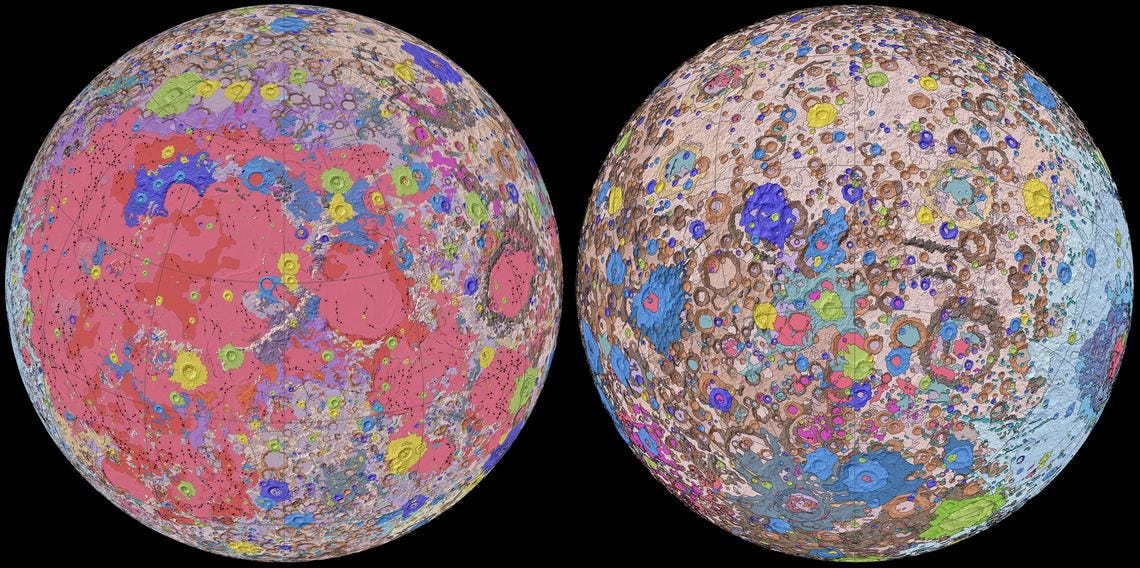
The map integrates new, high-resolution data from missions like the Lunar Reconnaissance Orbiter (LRO) and uses advanced terrain models to provide more accurate depictions of the Moon’s features. This seamless global map is useful for scientific research, future exploration missions, and educational purposes, enabling better understanding and resource planning for lunar exploration efforts.
ISRU: Building Lunar Infrastructure
Current research and technological capabilities are mostly focused on resources that can be extracted from the lunar regolith and used in-situ - for habitats, infrastructure, and life-support systems. These resources are essential for sustainable development of the lunar base, as delivering everything from Earth would be prohibitively expensive.
Water Ice
Water ice is perhaps the most critical resource for sustaining human presence on the Moon. Found primarily in permanently shadowed craters at the lunar poles, it offers a lifeline for life support and fuel production.
Studies suggest there could be approximately 600 million metric tons of water ice in the shadowed regions near the lunar poles. This amount could cover not only life support needs (drinking water and breathable oxygen) but also provide hydrogen and oxygen for rocket propellants, facilitating deep space missions.
Although there is strong evidence for the presence of water ice, many questions regarding its formation, distribution, and quality remain unanswered. Until rover missions collect more ground data, the potential for lunar ice extraction and utilization will remain uncertain.
Oxygen
Oxygen is crucial for life support and as an oxidizer in rocket propellant. Extracting oxygen from lunar materials is key to sustaining human activities on the Moon. It constitutes about 40-45% of the regolith by weight. Processing 1 cubic meter (approximately 1.5 tons) of regolith could yield about 630 kilograms of oxygen. This supports breathable air for astronauts and fuel for rockets, significantly reducing the need for resupply missions from Earth.
Silicon
Silicon is a fundamental element in electronics and solar energy technologies. The Moon’s abundance of silicon presents opportunities for in-situ production of solar panels and electronic devices.
Silicon dioxide makes up about 45% of the lunar regolith by weight, enabling the fabrication of solar cells and possibly microchips in the future. This could provide renewable energy and technological capabilities for lunar bases, fostering greater self-sufficiency.
Moreover, the Moon’s silicon resources can be utilized to manufacture mirrors and optical components, which have several critical applications:
Telescopes: Producing mirrors on the Moon allows for the construction of large-scale telescopes. The Moon’s lack of atmosphere eliminates atmospheric distortion, enabling clearer and more detailed observations of the Universe. Additionally, the far side of the Moon is shielded from Earth’s radio emissions and light pollution, making it an ideal location for both optical and radio astronomy. Lunar-based telescopes could revolutionize our understanding of the cosmos, allowing astronomers to observe distant galaxies, exoplanets, and cosmic phenomena with unprecedented clarity.
Solar Concentrators for Power Generation: Mirrors can be used to build solar concentrators that focus sunlight onto a small area to generate heat. This concentrated solar power (CSP) can drive turbines or other power generation systems, providing a robust and continuous energy source for lunar bases and industrial processes. CSP systems are particularly advantageous on the Moon due to the absence of atmospheric interference and the availability of uninterrupted sunlight during the lunar day (which lasts about 14 Earth days).
Industrial and Scientific Instruments: High-quality mirrors and lenses are essential for various instruments used in scientific research and industrial applications. These include spectrometers, laser systems, and optical communication devices. Manufacturing these components on the Moon reduces dependence on Earth imports and allows for the customization of equipment tailored to the lunar environment.
Metals
The lunar surface is rich in a variety of minerals essential for construction, manufacturing, and technological applications. Utilizing these minerals on-site could be essential for building and maintaining lunar infrastructure.
Iron is abundant in the form of iron oxide in lunar mare basalts, with iron oxide content ranging from 14% to 17% by weight. It can be used for constructing habitats, machinery, and tools, supporting a sustainable lunar presence.
Titanium, valued for its strength and low weight, has titanium dioxide concentrations varying from 1% up to 10% in some mare regions. It is useful for aerospace components and structural materials, enhancing the durability and efficiency of lunar equipment.
Aluminum and magnesium are key for creating strong, lightweight alloys; aluminum oxide and magnesium oxide constitute about 10-18% and 5-10% of the regolith by weight, respectively. These metals are essential for manufacturing components and structures, reducing the need to transport materials from Earth.
Rare Earth Elements (REEs)
REEs are vital for modern electronics, renewable energy systems, and high-tech applications. The Moon’s supply could help alleviate Earth’s limited and geopolitically sensitive reserves. Up to 50 parts per million (ppm) of REEs are found in KREEP-rich lunar regions, including elements like yttrium, lanthanum, cerium, and neodymium.
When examining market prices, REEs are significantly less valuable per kilogram compared to precious metals like gold and platinum group metals (PGMs). For instance, yttrium is priced at approximately $7 to $10 per kilogram, neodymium around $100 per kilogram, dysprosium about $400 per kilogram, and terbium approximately $800 per kilogram. The relatively low prices of REEs mean that the costs associated with their extraction and transportation from the lunar surface could outweigh the potential revenue.
However, while economic export potential remains a challenge, these resources could supplement terrestrial supplies, aiding in the production of electronics, magnets, and other critical technologies.
Thorium and Uranium
These radioactive elements could fuel nuclear reactors, providing a steady and potent energy source for lunar operations. Thorium, more abundant than uranium on the Moon, can be used in advanced reactors like Liquid Fluoride Thorium Reactors (LFTRs). Concentrations of thorium reach up to 5 ppm in certain lunar regions. Uranium, essential for conventional nuclear reactors, is typically found at levels less than 1 ppm in the regolith. While low concentrations may limit practicality for extraction, these elements could enhance energy independence on the Moon.
High-value Resources: Building Lunar Export Economy
Apart from resources that are essential for building and maintaining lunar infrastructure, there are several groups of resources that could be economically viable for Earth export.
Exporting high-value elements to Earth would boost the lunar economy and accelerate human expansion into space.
Helium-3
Helium-3 is a rare isotope on Earth but is relatively more abundant on the Moon due to solar wind implantation. It holds promise as a fuel for future clean and efficient nuclear fusion reactors. Between 1 to 5 million metric tons of helium-3 are embedded in the lunar regolith, according to theoretical models and analyses of Apollo mission soil samples. Just 25 tons could power the United States for an entire year (assuming fusion technology becomes viable), making it a potentially game-changing energy resource.
However, helium-3 presents significant risks and challenges alongside its promising prospects. The foremost concern is the market demand and the realistic timeline for developing helium-3-based fusion reactors. As of now, nuclear fusion technology is still in the experimental stage, and while progress is being made, a commercially viable fusion reactor—especially one utilizing helium-3—is likely decades away.
Worth noting that Helium-3 is not only a potential fuel for fusion energy but also has valuable applications in existing industries, reducing the risk associated with its extraction. It is used in cryogenics, neutron detection for nuclear security, and medical imaging, particularly in MRI lung imaging, where helium-3 enhances contrast and enables high-resolution scans of respiratory functions. These applications create a tangible market for helium-3 even in the absence of fusion breakthroughs, making lunar helium-3 mining a potentially viable enterprise with both short-term and long-term opportunities. While the demand remains relatively small compared to its fusion potential, these alternative uses provide a foundation for commercial viability and help mitigate investment risks.
Companies like Interlune are exploring the possibilities of helium-3 mining on the Moon. They are investigating the technological requirements, economic models, and strategic partnerships needed to make this venture feasible.
Precious Metals
Gold and platinum group metals (PGMs) are highly valuable on Earth due to their rarity and wide range of industrial applications, including electronics, catalysis, and jewelry. Their potential presence on the Moon has generated interest for possible extraction and export to Earth.
The presence of gold on the Moon is not well-documented, and existing data is largely speculative. Lunar samples returned by the Apollo missions have shown that gold is present in very low concentrations, typically less than 1 part per billion (ppb). This is significantly lower than average concentrations found in Earth’s crust.
Gold is one of the most well-known and valued precious metals, with a market value of approximately $60 million per metric ton. Its price fluctuates based on market conditions but generally maintains a high value due to its rarity, desirability, and diverse applications.
Platinum Group Metals (PGMs) include platinum, palladium, rhodium, iridium, osmium, and ruthenium. These metals are highly valued for their catalytic properties and are critical in automotive, electronics, and chemical industries.
Recent studies suggest that PGMs may be more abundant in lunar regolith than previously thought, especially in areas associated with ancient impact basins where asteroid material may have been deposited.
The Moon’s surface has been bombarded by asteroids and comets over billions of years. Since some asteroids are rich in PGMs and gold, it’s possible that impact sites on the Moon may contain higher concentrations of these metals.
Moreover, a large portion of asteroids impacting the Moon do so at relatively low heliocentric velocities, often below 10-12 km/s, which means that the energy of these impacts is insufficient to completely vaporize the incoming material. As a result, most of the asteroid’s material remains on the surface rather than rebounding into space, potentially contributing to deposits of valuable metals like platinum group metals (PGMs). However, these areas are not yet well characterized and would be challenging to identify and exploit.
Among the platinum group metals, Rhodium is one of the rarest and most valuable precious metals, with a market value of approximately $450M to $600M per metric ton, with an annual global supply of around 25 to 30 tons. Its price has been highly volatile, occasionally soaring above $800M per ton due to supply constraints and strong demand. Rhodium is primarily used in catalytic converters to reduce nitrogen oxide emissions in automobiles, making it crucial for meeting environmental regulations. It is also utilized in jewelry plating and as an industrial catalyst.
Palladium has a market value ranging from $60M to $75M per ton. In recent years, palladium prices have surged due to high demand and limited supply. Like rhodium, palladium is a critical component in catalytic converters for vehicles, helping to convert harmful gases into less dangerous substances. It is also used extensively in electronics, such as multilayer ceramic capacitors, as well as in dentistry and jewelry.
Iridium, valued at approximately $50M to $60M per ton, is notable for being one of the densest and most corrosion-resistant metals. Its market price remains relatively stable due to its limited applications and supply. Iridium is used in high-temperature materials, electrical contacts, crucibles for crystal growing, and as a hardening agent for platinum alloys.
For comparison, platinum has a market value of about $30M per ton. It is widely used in catalytic converters, jewelry, and various industrial applications. The high market values of these PGMs reflect their rarity and the critical roles they play in modern technology and industry.
Lunar Delivery Costs
The current state-of-the-art costs for delivering payloads to the Moon are exceptionally high, making the transportation of heavy mining machinery economically prohibitive. Companies like Intuitive Machines and Astrobotic Technology, operating under NASA’s Commercial Lunar Payload Services (CLPS) program, estimate delivery costs ranging from $1.2 million to $1.5 million per kilogram. These figures reflect the significant technical challenges and expenses associated with lunar missions using existing technology, effectively placing lunar mining out of reach with current cost structures. However, these costs are obviously prohibitive for any heavy machinery operations on the Moon.
In contrast, Elon Musk’s SpaceX is developing Starship, a fully reusable system aimed at dramatically reducing space transportation costs. Musk’s aggressive estimates suggest that, once fully operational, Starship could deliver 100-150 tons to the low Earth orbit (LEO) for less than $10 million per launch in 2-3 years, possibly down to $1 million in the long term. Moon missions, though, will require several such launches in order to refuel one Lunar Starship in orbit and send it to the lunar surface with 100 tons of usable payload. The number of refueling flights is a subject of debate, ranging from Musk’s early estimate of just four flights, to about 15 per NASA Artemis officials. These estimates, even with NASA’s 15 launches for $10M each per mission, put the delivery costs to the Lunar surface under $1500 per kg, and potentially less than $100 per kg in the long run, which is unprecedented by any means.
Return costs are harder to estimate, as current Artemis mission architecture assumes that Starship does not return back to Earth. Primarily designed as a Mars rocket, Starship can be fully refueled on Mars using methane and oxygen locally produced from martian atmosphere and water, driving return trip costs to as low as $200 per kg. The Moon is different: there is no atmosphere, and the regolith is low in carbon, making it difficult to produce methane. However, methane is only about 20% of the rocket propellant load by mass, the other 80% being oxygen that can be extracted from lunar regolith. With lunar gravity being 1/6th of Earth’s, a 100 ton lift back to Earth using Starship would only need about 50 tons of methane, pre-delivered from Earth. This would increase the return trip cost by about 50% compared to the earth-to-moon delivery, possibly more due to oxygen mining and refueling infrastructure, but still in the same order of magnitude. Even if it is several times higher than that, it should not significantly affect the economy of precious metal mining, as only mined and processed material, worth tens of millions per ton, will be returned back to Earth.
These projections are based on achieving full reusability, high launch frequency, and economies of scale. However, they remain speculative until the technology is proven and operational, and depend heavily on overcoming significant engineering and regulatory challenges.
Worth noting Starship is not the only option to return back to Earth. With no atmosphere and abundant solar energy on the Moon, using an electromagnetic railgun could be a more economic option to launch from the Lunar surface.
Moreover, storing precious metals on the Moon as financial assets instead of immediately returning them to Earth presents a unique opportunity to establish a lunar commodities reserve. Given their intrinsic value and global tradeability, these metals could serve as a foundation for a lunar-backed financial system. This approach would eliminate return transportation costs while creating a store of wealth in space, potentially attracting investment and enabling financial instruments such as lunar commodity futures, asset-backed lending, or even collateral for space infrastructure projects. Over time, as space commerce grows, these reserves could support a self-sustaining lunar economy, where metals are either extracted for in-space manufacturing or selectively transported to Earth based on market demand and optimal economic conditions.
Given the vast discrepancy between current costs and Musk’s optimistic projections, it’s prudent to analyze a range of delivery costs to assess the economic feasibility of lunar mining. For our analysis, we can consider a cost interval from $100,000 per ton (super-aggressive) to $10 million per ton (still optimistic but several times higher than target estimates). A reasonable average estimate of $1 million per ton provides a practical basis for further assumptions. This range allows us to explore both optimistic and conservative scenarios, helping to identify under what conditions lunar resource extraction might become economically viable.
Mining Equipment Mass and Cost
Terrestrial mining relies on massive equipment. For example, modern haul trucks, such as the Caterpillar 797F, have a nominal payload capacity of approximately 363 metric tons (400 U.S. tons) and a gross machine operating weight of about 624 metric tons.
Regarding ore grades, the average concentration of Platinum Group Elements in ores ranges from 5 to 15 parts per million (ppm). This means that to extract 1 kilogram of platinum, approximately 66 to 200 metric tons of ore need to be processed.
Therefore, if a haul truck moves 1 million metric tons of ore annually, and assuming an average ore grade of 10 ppm (10 grams per ton), this would result in the extraction of about 10 tons of platinum per year. With a truck mass of 624 metric tons, the equipment-to-platinum mass ratio would be approximately 62.4:1, meaning 62.4 tons of equipment are used per ton of platinum extracted annually.
Translating this to lunar mining amplifies the challenges. Transporting and operating hundreds of tons of mining equipment on the lunar surface would be extremely costly due to high space transportation expenses. However, in space, high delivery costs drive the need for mass optimization. This economic pressure encourages advanced engineering and material solutions to significantly reduce equipment mass. In our model, we explore equipment masses ranging from 1 to 100 times the mass of the resources extracted, which means that to mine 1 ton of resources we would need to use from 1 to 100 tons worth of equipment.
For equipment cost, we use an estimate of $100,000 per ton. This is conservative for bulk space hardware but higher than the cost of a modern high-tech car, suggesting that the mining machinery industry could achieve this price point through innovation and economies of scale.
Economics of Precious Metal Mining
With these considerations, we can approximately model the economics for potential mining of gold and most valuable PGMs depending on the mining equipment mass needed to extract 1 ton of resources, and equipment delivery costs.
For each metal, the area below the break-even curve represents conditions when mining could be profitable, i.e. the cost of mining equipment and transportation costs are less than market value of the resource.
While this model is very approximate and does not take into account all the cost factors like fixed infrastructure, operating costs, etc, it illustrates how reducing transportation costs from prohibitive levels to about $1 million per ton (or ideally less) could open up an economically viable zone where companies can innovate and build a compelling business case for lunar mining.
Rhodium seems the most interesting option, however, it will be challenging due to its scarcity - same as on Earth. As PGMs are mostly found together, it is reasonable to plan operations for several of them.
With our baseline assumption of equipment delivery costs at $1 million per ton, the prospects become even more encouraging. The analysis indicates that gold and the three most valuable PGMs—rhodium, palladium, and iridium—could be economically viable for lunar mining if the equipment mass is maintained under 50 tons per ton of mined material. This target is aligned with the equipment-to-resource mass ratios observed in terrestrial platinum mining. Achieving such efficiency on the Moon would require innovative engineering solutions, advanced materials, and optimized mining techniques tailored for the lunar environment.
These insights are inspiring and suggest that a lunar mining and export economy is indeed within the realm of possibility. The potential profitability of extracting high-value metals from the Moon could drive significant advancements in space technology, robotics, and sustainable mining practices. By pushing the boundaries of current capabilities, we open the door to not only accessing valuable resources but also to establishing a foothold for further space exploration and development.
Conclusion / Who is Doing Lunar Mining Now?
As the vision of lunar mining moves closer to reality, a growing number of companies are actively exploring the Moon’s potential. We are aware of around 100 organizations focused on utilizing lunar resources, and the community is expanding rapidly. In our next article, we will highlight the major players leading the charge in this exciting field, providing insights into their missions and innovations.
We invite anyone involved in lunar mining to join this conversation. If you represent a company working on lunar resource utilization or know of noteworthy organizations in this arena, please let us know. Your contributions are invaluable as we aim to provide a comprehensive overview of the industry’s landscape.
References for Further Reading
Lunar Resources:
• NASA Lunar Exploration Program Overview
• U.S. Geological Survey (USGS) Reports on Lunar Resources
Lunar Transportation
• Astrobotic Technology: Payload User’s Guide
• SpaceX Starship Updates: SpaceX Official Website
• Elon Musk’s Presentations:
• 2020 Mars Society Convention
• Intuitive Machines: Payload Services
None of the above constitutes investment advice.
This explains EVERYTHING! 😁👏🏽👏🏽👏🏽💫💫💫
A very comprehensive analysis of the potential of the lunar economy, from a trusted team. Thanks for sharing!