Understanding Gravitational-Wave Observatories: A Pillar of Modern Fundamental Science
Find out why funding fundamental science often represents a relatively budget-friendly method for enhancing our technological capabilities
Issue 80, Subscribers 11,223. Featuring Professor Denis Martynov, School of Physics and Astronomy at the University of Birmingham.
The Laser Interferometer Gravitational-Wave Observatory (LIGO) is a monument to human curiosity and ingenuity. Built to detect gravitational waves — ripples in the spacetime predicted by Einstein's general relativity, LIGO has not only confirmed a major scientific theory but also laid the ground for future advances in various technological fields. Understanding the impact of this and other existing and planned gravitational-wave detectors requires delving into the specifics of these projects and their broader implications for technology and the economy.
Project Overview and Metrics
The detection of gravitational waves is crucial for fundamental physics as the direct confirmation of General Relativity. Gravitational waves also provide a new way to observe and understand the most violent and energetic events in the universe. The first direct observation of gravitational waves was made in September 2015 by the Advanced LIGO observatories, detecting gravitational waves with wavelengths of a few thousand kilometers from merging a couple of black holes. This discovery was awarded the Nobel Prize almost immediately - in 2017.
LIGO is composed of two enormous laser interferometers located in Livingston, Louisiana, and Hanford, Washington. Each facility houses an L-shaped setup where laser beams travel 4 kilometers along two perpendicular tubes, measuring minute distortions caused by passing gravitational waves. The sensitivity of these instruments is astonishing; they can detect changes in length smaller than one ten-thousandth the diameter of a proton.
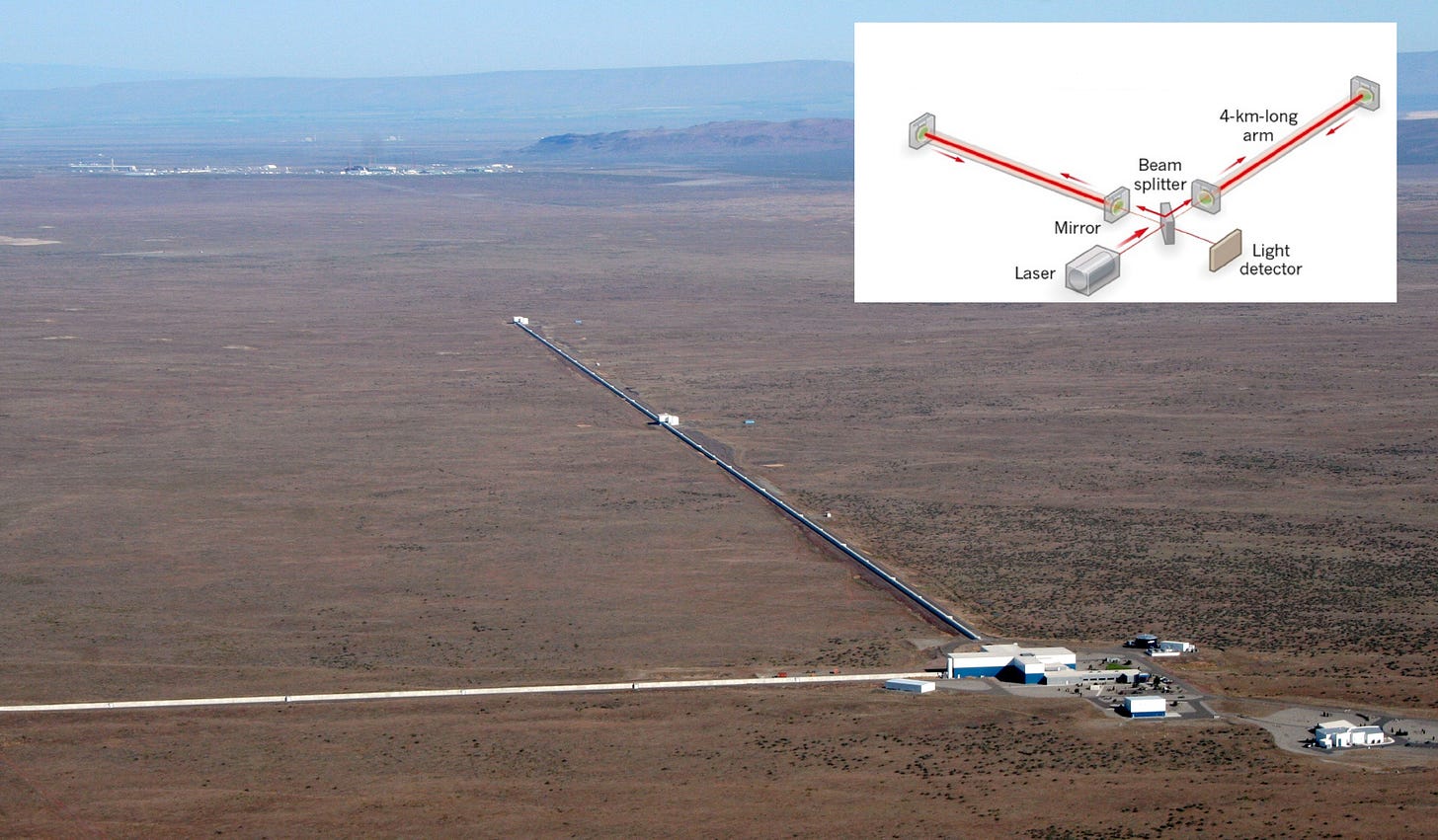
The project's scale and precision are supported by a budget that reflects its ambitious goals. The initial construction of LIGO was funded by the National Science Foundation (NSF) with an investment of approximately $1.1 billion, making it one of the most expensive projects in the history of fundamental science. This funding covers not only the construction and maintenance of the facilities but also the extensive research and personnel costs involved in the project.
The Now and Future of Gravitational-Wave Astronomy
LIGO is not alone in the field of gravitational-wave astronomy. The Virgo interferometer, a collaboration among several European countries located near Pisa, Italy, is an integral component of the global network of gravitational-wave observatories. Like LIGO, Virgo is equipped with a large-scale interferometer design featuring arms that are 3 kilometers long—slightly shorter than LIGO's 4-kilometer arms. Since becoming operational in 2007, Virgo has played an important role in the multi-detector observations of gravitational waves, notably during the landmark detection of gravitational waves from a binary neutron star merger in 2017. The construction and successive upgrades of Virgo have incurred costs of approximately €170 million, with further enhancements planned.
In addition to Virgo and LIGO, the KAGRA observatory in Japan adds another layer of depth to the international gravitational wave detection effort. Located in the Kamioka mine, KAGRA features an underground design with 3-kilometer-long arms, similar in scale to Virgo. The collaborative efforts of American (LIGO), European (Virgo), and Japanese (KAGRA) observatories are crucial for improving the accuracy and reliability of gravitational wave detections. By combining measurements from these geographically dispersed detectors, scientists can triangulate the sources of gravitational waves more precisely.
Looking ahead, the European Einstein Telescope (ET) represents the next generation of ground-based gravitational-wave observatories. ET will have three arms each 10 kilometers long, arranged in a triangular configuration. This design enhances by a factor of 10 its ability to detect and pinpoint the sources of gravitational waves, compared to LIGO and Virgo. The estimated cost for this ambitious project is around €2 billion, with construction hoped to begin in the late 2020s and operations starting in the 2030s (compare the cost to that of the James Webb Space Telescope at ~ $10 billion). Cosmic Explorer is another example of a proposed third-generation ground-based gravitational wave observatory. It will employ an L-shaped layout similar to the LIGO detectors but with arms that are ten times longer, each stretching 40 kilometers.
The Laser Interferometer Space Antenna (LISA) is an even more ambitious project, intended to be the first space-based gravitational-wave observatory. A collaboration primarily led by the European Space Agency (ESA) with contributions from NASA, LISA will consist of three spacecraft arranged in a triangular formation, each separated by 2.5 million kilometers. LISA aims to detect gravitational waves at low frequencies that are inaccessible to Earth-based observatories, particularly from supermassive black hole mergers and other exotic events in the cosmos. The anticipated launch date for LISA is in the early 2030s, with the project cost estimated at approximately €1.5 billion.
Economic and Technological Benefits of Gravitational-Wave Observatories
While the direct applications of detecting gravitational waves might seem esoteric, the indirect benefits of projects like LIGO are broad and significant. They act as beacons of innovation, developing technologies that find uses in fields that require similar precision and environmental control. For example, the ultra-high vacuum systems and vibration isolation techniques developed for these projects have potential applications in sectors ranging from manufacturing to aerospace. By advancing the state of the art in laser technology and quantum measurement, LIGO prepares us for technologies such as quantum computing.
Consider the technology of squeezed light as another example. It has been crucial in enhancing the sensitivity of LIGO and holds significant potential for advancing quantum technologies. Squeezed light involves reducing quantum noise in one property of light (like its phase) at the expense of increasing it in another (like its amplitude), which is key for measurements at or beyond the standard quantum limit. In quantum technologies, this principle can improve the precision of quantum measurements and sensors used in quantum computing. The use of squeezed light in quantum cryptography could lead to more secure communication channels that are less susceptible to eavesdropping. This cross-application of squeezed light from astrophysics to quantum technologies exemplifies how innovations in fundamental science can propel advancements in emerging technological fields.
Professor Denis Martynov from the School of Physics and Astronomy at the University of Birmingham provides another valuable example:
Neutron stars are incredibly dense objects, with densities exceeding that of atomic nuclei. During their formation and subsequent evolution, neutron stars undergo extreme compression, leading to densities that push the limits of our current understanding of nuclear physics. Gravitational waves emitted during neutron star mergers provide a direct probe of these extreme conditions. Unlike electromagnetic radiation (such as light or X-rays), gravitational waves are not absorbed or scattered by matter. This means they can travel through the universe without being significantly affected by intervening material. As a result, gravitational waves offer a clean signal that is not obscured by intervening matter, allowing scientists to directly observe the dynamics of dense matter in neutron stars without interference.”
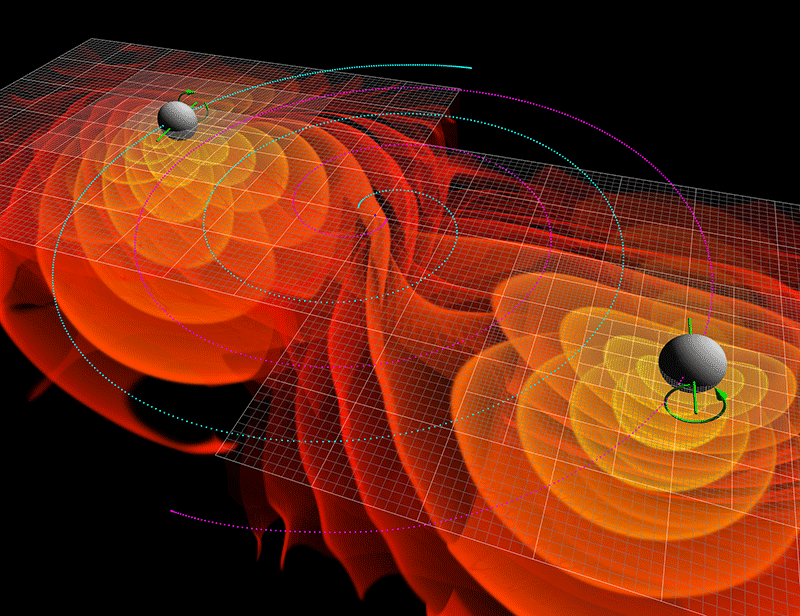
Educational Analogy: How Fundamental Science Projects Serve as “Universities” for STEM Community
One might wonder why we develop these technologies within a grand-scale fundamental science project rather than directly within their intended applications. The answer lies in the market readiness of these applications. Achieving technological readiness within the context of groundbreaking international collaborations and mega-projects can dramatically shorten the time to market for innovations when they become economically viable. Funding fundamental science often represents a more budget-friendly method for enhancing our technological capabilities. Take, for instance, the total investment in LIGO, which is about $1.1 billion, compared to similar amounts poured into tech ventures such as Bird ($0.9B), QuantumScape (>$1.5B), and Rigetti (>$0.65B), all of which are still in the process of developing their products or finding their market fit or bankrupt. Furthermore, in 2023 alone, $27 billion in venture capital was invested in 3,200 startups that eventually failed.
Consider the example of superconductors: while these materials have been in the market for some time, promising virtually loss-free transmission of electricity and enabling technologies like levitating trains, their high costs have so far limited their applications primarily to specialized fields such as particle accelerators. However, as the technology matures and costs decline, we can expect broader adoption across various sectors, illustrating the long-term potential of investments in foundational research.
Much like how a university education broadens a student's horizons and prepares them for unforeseen career challenges, fundamental research projects prepare the technological landscape to meet future unforeseen needs and opportunities. Each discovery and technological advance to a repository of knowledge that future scientists and engineers can draw upon, enabling rapid adaptation and innovation when new challenges and opportunities arise in the economy.
In conclusion, while the immediate practical applications of gravitational-wave astronomy might not be apparent, its long-term contributions to technological development and economic resilience are profound. By investing in fundamental science, we lay the groundwork for future technological revolutions, ensuring that we remain at the cutting edge of innovation and prepared for the challenges of tomorrow. Who knows? Perhaps this fundamental research will one day help humanity travel between galaxies or understand the fundamental principles necessary to develop new materials with unique properties. What do you think about this topic? Please, shoot us an email via hello@spaceambition.org or leave a comment below.