Current Status and Purpose of Exoplanet Exploration
In the last decade, over 5,500 exoplanets have been discovered, some with known atmospheric compositions. Google and others have funded these efforts, showcasing their significance beyond science.
Issue 71. Subscribers 11 063.
Exciting news! Gain exclusive access to the behind-the-scenes research fueling our newsletter, featuring the latest on 2024 space conferences and space tech investors. More information here.
The quest to discover exoplanets, celestial bodies orbiting stars beyond our solar system, marks a pivotal chapter in modern astronomy. An exoplanet, simply put, is a planet not in our solar system, orbiting a certain massive central object: a star, a brown dwarf, or a stellar remnant (e.g. a neutron star). These objects are lighter than the threshold for thermonuclear fusion of deuterium (roughly below 13 Jupiter masses), and have a mass ratio with their central object below less than 1/25, defining them as planets regardless of their formation process.
Exoplanets are incredibly diverse, ranging from 'Hot Jupiters' - massive planets close to their stars, to 'Super-Earths' and 'Mega-Earths' - larger than Earth but smaller than ice giants, and even 'Ocean Worlds' and 'Chthonian Planets', ‘Puffy Planets’, etc, each with unique characteristics and environments.
The speculation about the existence of planets orbiting other stars dates back as far as the 16th century. However, the first evidence of an exoplanet was noted in 1917, but that planet candidate was not recognized as such until 2016. The first confirmed detection of an exoplanet came in 1992 with the discovery of terrestrial-mass planets orbiting the pulsar PSR B1257+12 located 2,300 light-years (710 parsecs) from the Sun. These planets were detected by astronomers Aleksander Wolszczan and Dale Frail at the Arecibo Observatory in Puerto Rico. They used a method known as pulsar timing - read more about this and other methods in the devoted section below.
The first planet discovered around a sun-like star (let’s say it simply, a regular star) was found in orbit of 51 Pegasi in 1995 by Swiss astronomers Michel Mayor and Didier Queloz. This discovery was made using the radial velocity method, which measures variations in the speed of the star as it moves toward or away from Earth due to the gravitational pull of the planet. Together with the Big Bang theorist James Peebles, Mayor and Queloz were awarded the Nobel Prize in Physics in 2019 “for their contributions to our understanding of the evolution of the universe and Earth's place in the cosmos.” This recognition underscores the fundamental importance of exoplanet research: it's not just about finding new worlds; it's a quest to understand our universe's vast complexity and our place within it. For our readers, the progress in exoplanet research offers a glimpse into future technologies and hence potential new markets.
The field of exoplanet research surged forward in 2006, propelled by advances in radial-velocity methods and the SuperWASP project. Let's dive deeper into the methods and tools that caused that technological leap and the subsequent launch of orbital instruments dedicated to planet search that enabled the detection of distant worlds with greater accuracy and frequency.
Techniques for Exoplanet Discovery
First to mention, Direct Imaging is the method that involves capturing images of exoplanets by blocking the overwhelming light from their parent stars. Basically, it’s direct photography of planets, which is a significant technical challenge due to the faintness of planets compared to stars. Instruments like the Gemini Planet Imager and VLT-SPHERE are designed for this purpose, although they primarily image large, distant gas giants.
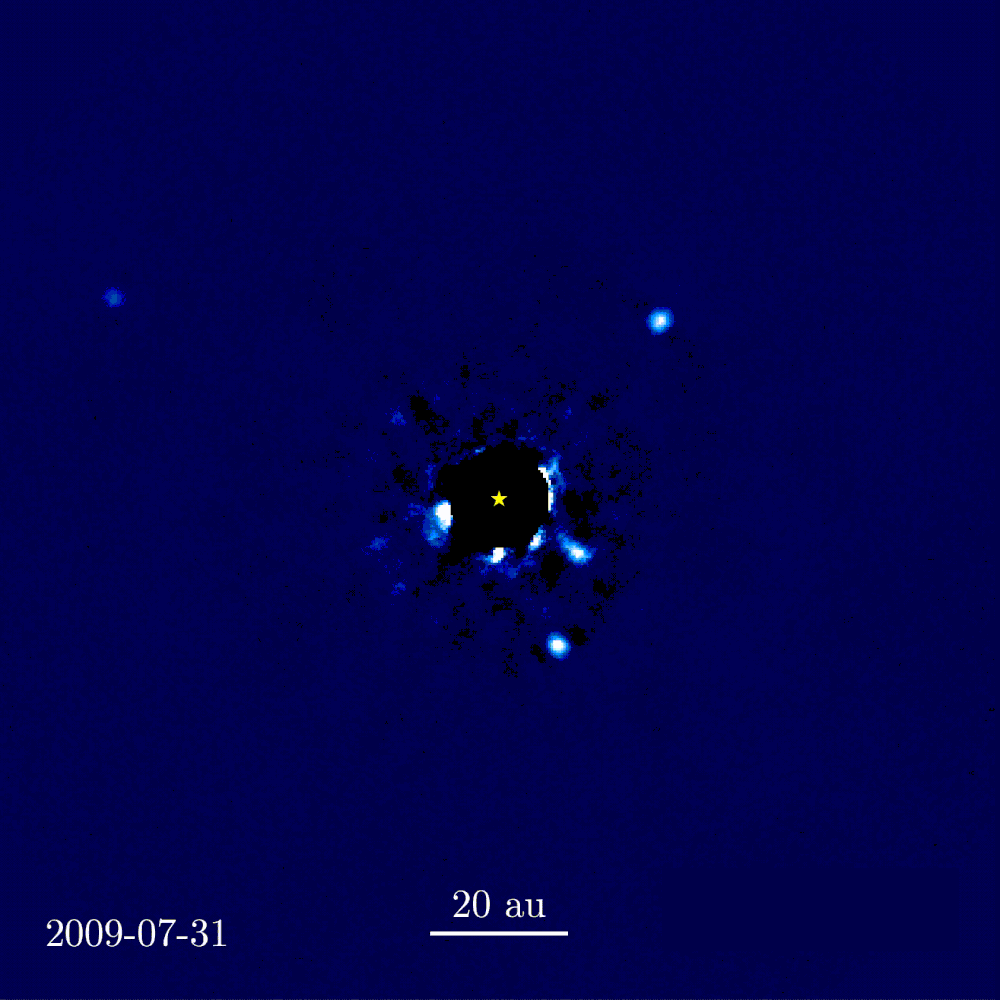
Below you will find a short and not complete summary of the indirect methods (there are a plethora of them, so listing and detailed description of each deserves a separate article). Each of these methods has its strengths and limitations, and they are often used in conjunction to confirm the existence and characteristics of exoplanets.
Transit Method: Utilized by missions like Kepler, this method detects exoplanets when they transit across their star, causing a slight dimming. The extent of dimming reveals information about the planet's size and orbit. This method is most effective for planets whose orbits are aligned with our line of sight. This method requires a specific alignment: the orbital plane of the planet needs to be nearly edge-on from our line of sight. At first glance, it might seem that only a small fraction of exoplanets could be detected this way because such alignments would be rare. However, the huge number of stars observed, combined with the higher probability of detecting close-in planets and sophisticated data analysis techniques, makes it a highly successful method for detecting exoplanets.
Radial Velocity (Doppler Method): This technique detects exoplanets by observing subtle changes in the movement of a star, caused by the gravitational pull of an orbiting planet (or several planets). As the planet orbits, it exerts a gravitational pull, causing the star to wobble slightly around a common center of mass. This is similar to how both an adult and a child, while spinning around each other, actually move around a point between them, with the child moving faster due to its smaller size. Even though the star's movement is less pronounced compared to the planet's, the star is much brighter, so one can detect it through changes in the star's light spectrum due to the Doppler effect: when a star moves towards us, the light waves it emits are compressed, shifting towards the blue end of the spectrum (known as a "blue shift"). Conversely, when the star moves away from us, the light waves are stretched, shifting towards the red end of the spectrum (a "red shift"). This method is particularly effective for finding planets whose orbits don't line up in a way that would allow one to use the transit method.
Transit Timing Variation (TTV) and Transit Duration Variation (TDV): TTV and TDV are methods used to detect the presence of additional planets in a system by observing variations in transit timing and duration. Simply put, astronomers could have detected the first planet near a star by the transit method. Continued observations could reveal variations in transit timing that are not typical for an elliptic orbit of this planet. These variations can be caused by gravitational interactions between planets, revealing information about non-transiting planets or multiple star systems.
Gravitational Microlensing: This method relies on the gravitational field of a star acting as a lens, magnifying the light of a distant background star. Planets orbiting the lensing star cause anomalies in magnification, making this method sensitive to planets at distances of 1–10 AU (astronomical units) from their stars.
Astrometry: If you were already amazed by our civilization's ability to discover planets using the direct method, prepare to be even more astonished by the wonders of astrometry. Astrometry is the science of precisely measuring a star's position in the sky and monitoring any changes over time due to the gravitational pull of its planets. Unlike the radial velocity method, which focuses on measuring velocity, astrometry allows us to directly detect (or, simply put, to see with the help of a telescope) the subtle movements of a star caused by the gravitational influence of an orbiting planet. Although the scale of these motions is quite small, making them challenging to detect, astrometry remains a powerful tool in our celestial toolkit.
Pulsar Timing: A pulsar is the compact, highly dense remains of a star that has undergone a supernova explosion, becoming a neutron star. These pulsars emit radio waves with remarkable regularity during their rotation. The inherent consistency in a pulsar's rotation means that even minor irregularities in the timing of its radio pulse emissions can serve as indicators to monitor the pulsar’s movements, caused by nearby planets. It was through this method that the first confirmed discovery of an extrasolar planet was made.
Notable Exoplanet Discovery Projects and Milestones
SuperWASP: The UK's Wide Angle Search for Planets (SuperWASP) is a pivotal project in the exoplanet search. Established with £1 million in setup costs, it has become a global leader in ground-based transit searches, discovering over 192 exoplanets. Both SuperWASP-North and the newly built WASP-South instruments opened for observation in early 2006. Despite the program’s low cost, Its achievements have been recognized with awards like the Royal Astronomical Society's Group Achievement Award in 2010.
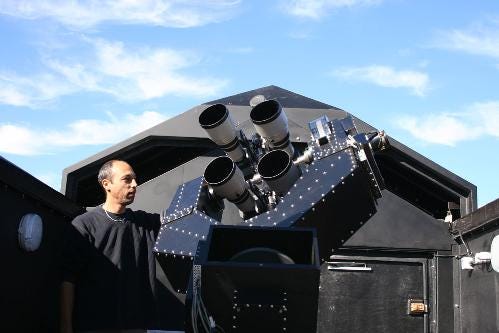
Kepler Space Telescope: NASA's Kepler has been a game-changer in exoplanet discovery. Despite a life-cycle cost of around $600 million, it identified 2,778 exoplanets (out of 5603 total, 31st of January 2024), making it one of the most successful missions in this area. Notable discoveries include Earth-sized planets in habitable zones and the first planets observed transiting the same star.
Transiting Exoplanet Survey Satellite (TESS): Launched to its highly elliptical orbit by Falcon 9 in 2018, TESS's achievements include mapping over 93% of the sky and confirming 329 exoplanets. It was initially a privately funded mission with support from institutions like the Kavli Foundation and Google. Its total costs are reported to be $378 million.
CoRoT: The Convection Rotation and Planetary Transits (CoRoT) space telescope, led by the European Space Agency with a total cost of approximately $250 million, operated from 2006 to 2013. It discovered 34 exoplanets, focusing on confirmed and fully characterized planets rather than candidate lists.
Canadian MOST Project: The Microvariability and Oscillations of Stars (MOST) was Canada’s first space telescope, launched in 2003 at $10 million. This project paved the way for future exoplanet research and laid the foundations for subsequent discoveries.
Five Hundred Meter Aperture Spherical Telescope (FAST): Completed in 2016 with a budget of $175 million, FAST is one of the largest radio telescopes in the world, contributing significantly to astrophysical studies including exoplanet research with pulsar timing method.
By the way, in one of our previous articles, we explored various telescopes that are not specifically dedicated or designed for exoplanet studies. Interestingly, these telescopes can sometimes play a role in the discovery or confirmation of exoplanets. A prime example of this is the multi-planet system orbiting the star HR 8799. This system was initially identified in 2008 through direct imaging by the Keck and Gemini observatories. However, it was later realized in 2009 that the Hubble Space Telescope had captured an image of one of these planets, HR 8799 b, back in 1998. This discovery hints at the exciting possibility that further analysis of Hubble's photographic archives could unveil more hidden exoplanets.
Upcoming Directions and Strategies in Exoplanet Exploration
The frontier of exoplanet research is set to expand dramatically, driven by ambitious projects and cutting-edge technologies.
The James Webb Space Telescope (JWST), already in operation, with an estimated cost of around $10 billion, is designed to observe the infrared universe like never before. Its capabilities are expected to enable the study of the atmospheres of exoplanets in detail, potentially identifying chemical signatures of life. In January 2023 it already confirmed its first exoplanet. With the ability to provide the highest fidelity spectrum of exoplanets, this instrument sets promise for astrobiology's future.
PLATO (PLAnetary Transits and Oscillations of Stars): This European Space Agency mission, with an estimated budget of €850 million, aims to launch in 2026. PLATO’s objective is to discover and study Earth-like exoplanets around Sun-like stars, focusing on their habitability.
ARIEL (Atmospheric Remote-sensing Infrared Exoplanet Large-survey): Slated for a 2028 launch, ARIEL, with a budget of around €550 million, is dedicated to studying the atmospheres of a diverse sample of exoplanets, which will help in understanding the physics and chemistry of these distant worlds.
The Extremely Large Telescope (ELT): The ELT, costing approximately €1.5 billion, is expected to be operational by the late 2020s. Its 39-meter primary mirror will make it the largest optical/near-infrared telescope in the world, providing unprecedented views of potentially habitable exoplanets.
Nancy Grace Roman Space Telescope: Previously known as WFIRST, with an estimated cost of $3.2 billion, this NASA mission, planned for the mid-2020s, aims to study dark energy, exoplanets, and infrared astrophysics. It will include a coronagraph instrument to directly image exoplanets.
Ground-Based Observatories: Next-generation ground-based observatories, such as the Giant Magellan Telescope and the Thirty Meter Telescope, each costing over $1 billion, are expected to contribute significantly to exoplanet research. These telescopes will offer enhanced capabilities for characterizing exoplanet atmospheres and searching for potentially habitable planets.
Closeby Habitable Exoplanet Survey (CHES): This upcoming mission aims to discover Earth-like exoplanets in nearby star systems using cutting-edge detection methods.
Earth 2.0 (ET) Mission: Focused on finding Earth analogs, the ET mission represents China's ambitious goals in the field of exoplanet research.
These upcoming projects not only represent significant investments in the field of astronomy but also symbolize the continued commitment to understanding our place in the universe. With state-of-the-art technology and ambitious goals, the future of exoplanet research is aimed at unlocking many of the cosmos' mysteries.
The Influence of Exoplanet Discovery
The influence of exoplanet science extends beyond academic curiosity, impacting various economic sectors. We demonstrated in one of our earlier articles that technology developed for space missions often trickles down to other industries. High-precision optics used in telescopes can enhance everything from photographic equipment to laser engineering. The data processing techniques developed for analyzing vast amounts of astronomical data have applications in sectors like finance, where similar approaches can be used for risk assessment and market analysis. Moreover, the push for more sophisticated space missions stimulates the aerospace industry, creating demand for advanced materials, electronics, and engineering solutions.
However, we must admit that the search for new worlds, which we will not reach even within the next several centuries, seems less attractive for a future sustainable economy than, for example, the exploration of Mars or space tourism.
In the case of exoplanets, we follow the subtle twinkling of stars to, using intricate and indirect measurements, perceive new worlds in incredible detail: not only the mass and size of the planet, the distance to the parent star, but also vast oceans, molten lava, and atmosphere composition are often revealed in these twinklings. Why should we be able to spend huge budgets on such details? The answer to that often lies on the surface, but escapes sight: you need to be able to do something accurately and carefully to praise the scientific method.
Millions of students encounter astronomy during their studies, and even though many shift their careers towards various sectors in the real economy, along with the thousands of dedicated astronomers, they play a crucial role in reinforcing public trust in the scientific method. They significantly ease the task of persuading the public about critical issues like global warming and the urgency of addressing them. The rationale is straightforward: if we possess the capability to decipher the atmospheric conditions of distant exoplanets, it stands to reason that we should have a profound understanding of our own planet's atmosphere.
The inspirational aspect of exoplanet research cannot be underestimated. The quest to find new worlds and possibly life beyond Earth can drive public interest in science and technology, potentially influencing education and career choices. This leads to a more scientifically literate populace and a workforce skilled in high-tech sectors, which is essential for modern economies.